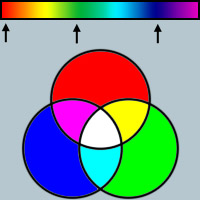
As you probably know... a given color of light can generally, be described by one number (e.g., the frequency of its wave length).
The color white, however, has no associated frequency; it is composed of equal amounts of all the (visible) frequencies of light. Black, of course, is the absence of light. And various shades of grey are generally composed of various intensities of white (as you lower the overall intensity of white, the grey gets darker and approaches black).
The question is: why is light (optically) additive? More specifically, why is it that when we add Red and Green together we get Yellow; Green and Blue together, we get Cyan; Red, Green, and Blue together, we get white?
(Please don't confuse this with the subtractive quality of pigments/paints, where as we add more colors, particularly more of the primary colors red, yellow, and blue, we approach black.)
Could we use three (or more) different colors and achieve the same result?
The problem is not one of physics, but of biology (human physiology in particular). The retina contains light sensitive cells. Some, called rods, are sensitive to low light levels and are not differentiated by color sensitivity, and so allow you to see in black and white in low illumination. Other receptor cells, called cones, come in three varieties, "red sensitive", "green sensitive" and "blue sensitive". These categorizations are oversimplifications, as each has a response curve of different degrees of sensitivity to various wavelengths of light, and in fact overlap considerably.
So let's not oversimplify to that great a degree, but simplify enough so we can use some concrete numbers.
Let's say the responsiveness of the three types of cell to some distinct wavelengths of light are as follows:
Light "red-sens cell" "green-sens cell" "blue-sens cell"
7000-red 6 4 0
yellow 5 5 1
green 4 6 2
cyan 2 7 4
4500-blue 1 6 5
--------
so that if monochromatic (single wavelength) yellow light hits the retina, the R, G and B cells will respond in a ratio of 5:5:1. But if a mix of monochromatic red and monochromatic green falls on the retina the response ratio will be 6+4:4+6:2 = 10:10:2 = 5:5:1, which is the same as for the monochromatic yellow light.
A similar scheme would work for cyan from blue and green. It's likely that other well-spaced sets of three wavelength could serve as primary colors, but it's also likely that the choice of r,g and b is somewhat optimal given the spectral response characteristics of the three types of cone, and is able to reproduce more wavelengths of monochromatic light than other combinations. (Nothing guarantees being able to reproduce exactly the 3-level cell response ratios of all wavelengths of monochromatic light.)
In practice, most light is not monochromatic, nor even consisting of line spectra (a select group of distinct wavelengths), but rather exhibits a continuous spectrum. If the visible range of wavelengths is present in approximately equal proportions, all the cones will be stimulated equally and we'll see white or gray depending on the illumination level. It's a philosophical question as to whether everyone sees this the same way; but whatever way we see it, that is what we've been taught is called "white". It is actually more complicated than this as the brain interprets
differences in the response ratio from one part of the retina to another. So, for example if all the cones are stimulated equally at level 3 in one patch on the retina and at level 6 in another patch, it's likely the former will be perceived as gray and the latter as white. Then at another time when again all the cones in one patch are equally responding at level 6, and in another patch at level 12, the level-6 area this time will be perceived as gray as the level-12 area now "looks" white. In this manner, objects maintain a color constancy even when viewed in bright light or dim light.
Some characteristics of light can play tricks on the color sensing of the eye-brain combination. Most fluorescent lights have notable line spectra rather than continuous spectra. The spectral lines (mix of distinct frequencies) are sufficiently spread so the light looks white, but objects viewed under them may have irregular reflecting spectral properties, and the wavelength of good reflection might not always correspond to the distinct wavelengths in the light, and so the object could look a different color under fluorescent from the way it looks under a continuous spectrum such as sunlight.
Also, for example, the moon looks really bright even though it's dark rock, since is in bright sunlight even though it is night time (usually) and you are looking against a dark background. The brain is again comparing relative levels and understanding the moon to be bright.
Certainly if more than 3 primaries were used, it would not hurt the approximation process to the color matching. In fact, if the entire reflectance or radiation spectrum of a given patch could be duplicated exactly, the eye would have no way of discerning it from the original.
I understand that birds may have four different color sensors, and so would need at least four primary colors, but more wouldn't hurt either.
Edited on March 4, 2004, 3:06 pm
Edited on March 4, 2004, 3:06 pm
|
Posted by Charlie
on 2004-03-04 15:02:25 |